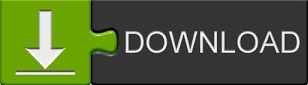
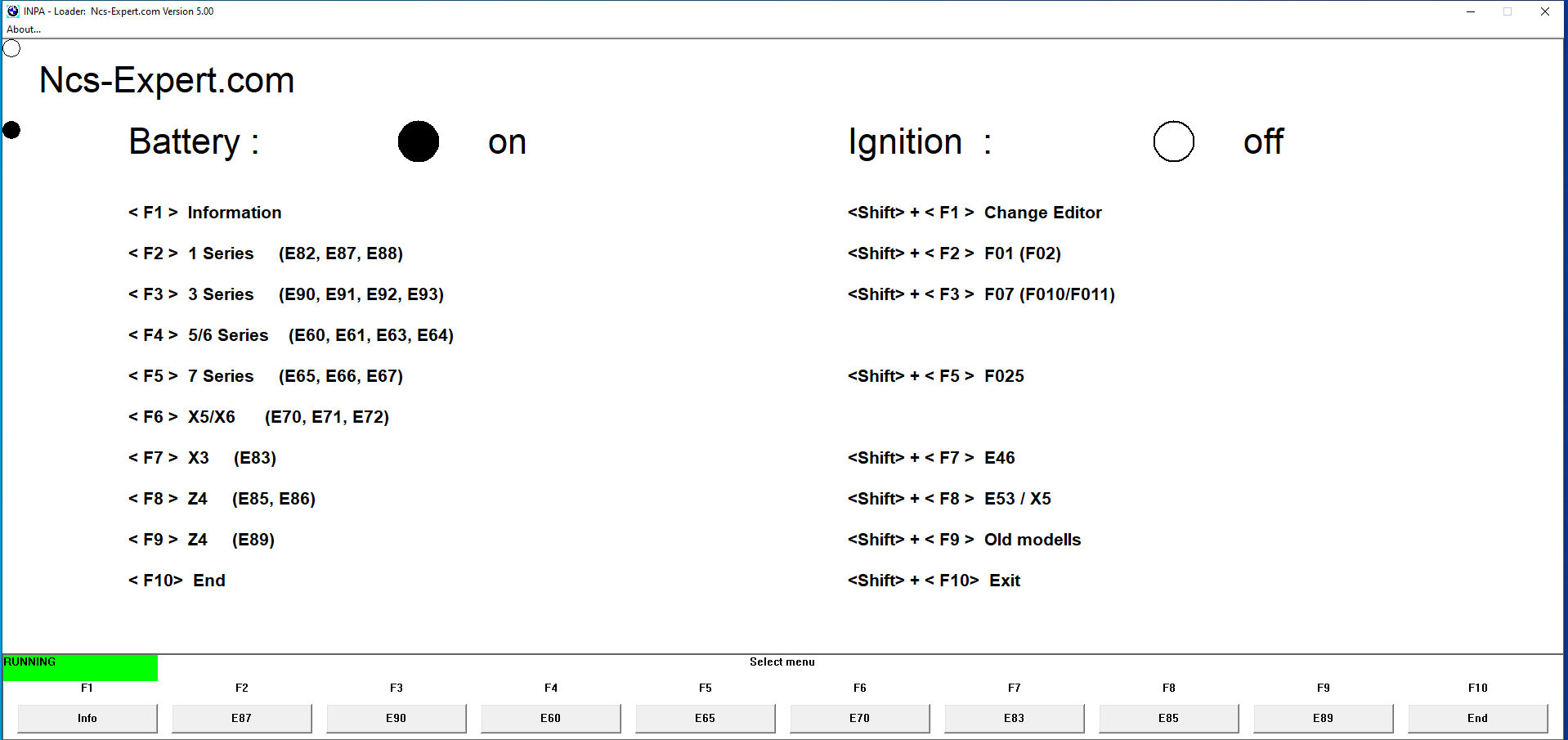
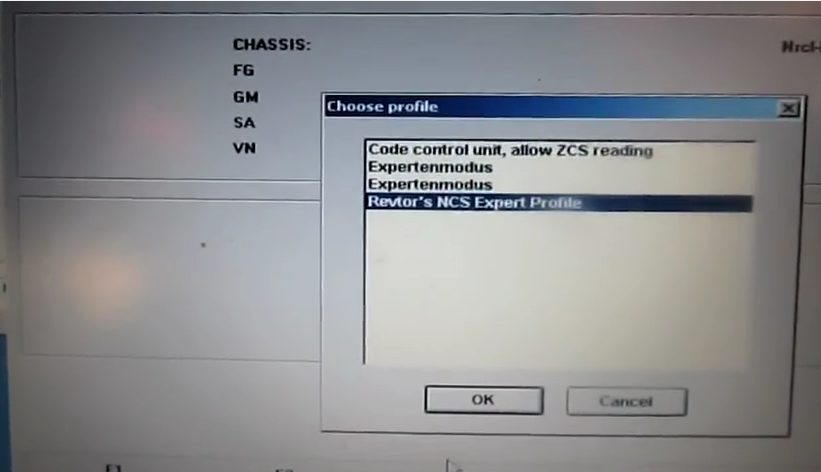
Structural tools such as X-ray diffraction (XRD) provide information about the crystal symmetry and lattice spacing, but due to nanocrystal size broadening effects, structural subtleties such as strain and alloying remain hidden.

Optical methods such as ultraviolet/visible (UV/vis) spectroscopy and photoluminescence (PL) are indispensable, but without much a priori knowledge about the heterostructure, the assignment of spectroscopic features can be ambiguous. (14,15)ĭespite the myriad of synthetic and theoretical approaches that have recently been put forward to control the properties of heterostructured nanomaterials, their internal atomic-scale characterization remains challenging. In fact, the wave function engineering approach in CSQDs (10,11) that makes use of epitaxial strain and alloying through ion exchange reactions has led to, for example, enhanced photoluminescence quantum yields, (5,6) reduced charge carrier recombination, (12,13) and their use as luminescent solar concentrators. Additional control over the spatial distribution patterns of charge carriers is achieved by varying the structure of the heterojunction itself, that is, making it epitaxial and sharp or alloyed and smooth.
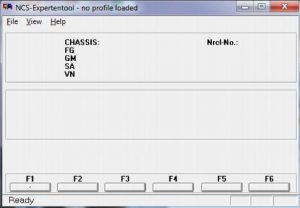
In type II structures, the band energies have a staggered band alignment that can result in separation of carriers across the material interface, benefitting charge extraction toward the electrodes in a photovoltaic device. In type I heterostructures, the band gap of one material is encompassed within the band gap of the other material, which confines excited charge carriers (electrons and holes) exclusively within the material containing the smaller band gap. This can lead to two main types of band alignment: type I and type II. (4−9) Compared to pure-phase QDs, core/shell QDs (CSQDs) exhibit two additional degrees of freedom for tuning the energetics of the nanoparticle: the shell material and thickness. (1−3) Optical and electronic properties can be further tailored when two or more semiconducting materials are put together, forming a QD heterostructure. Their tunability and synthetic flexibility make them ideal candidates for applications in solar energy harvesting, biomedical imaging, photocatalysis, light-emitting devices, and quantum computing. This study highlights the challenges with synthesizing II–VI quantum dot heterostructures and the power of XAS for understanding the internal structure of heterogeneous nanoparticles.Ĭolloidal semiconductor nanocrystals, that is, quantum dots (QDs), exhibit size- and shape-dependent optoelectronic properties due to their quantum-confined (excited) states. Our structural analysis is extended with electronic band structure calculations and UV/vis absorption spectroscopy, demonstrating that the alloyed Cd xZn 1– xTe/CdSe core/shell quantum dots exhibit a direct band gap, different from the predicted type II band alignment of the intended ZnTe/CdSe core/shell quantum dots. We synthesize particles of different shell thicknesses and performed synthetic control studies that allowed us to discard a ZnTe/CdTe/CdSe core/shell/shell structure and confirm the alloyed core/shell structure. Our multimodal characterization approach confirms (1) the presence of Cd–Te bonds, (2) cation alloying in the particle core (and the absence of anion alloying), and (3) a patchy pure-phase CdSe shell. We combine our XAS results with transmission electron microscopy (TEM) sizing and elemental analyses, which allows us to propose models of the internal particle structure. We employ X-ray absorption spectroscopy (XAS) at all four K-shell ionization edges (Zn, Te, Cd, and Se) and perform global fitting analysis to extract the first-shell bond distances. This work shows that ZnTe/CdSe core/shell quantum dots synthesized by a standard literature procedure in actuality have an alloyed Cd xZn 1– xTe core.
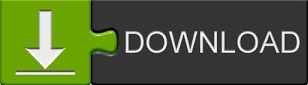